- Undergraduate
Bachelor's Degrees
Bachelor of ArtsBachelor of EngineeringDual-Degree ProgramUndergraduate AdmissionsUndergraduate Experience
- Graduate
Graduate Experience
- Research
- Entrepreneurship
- Community
- About
-
Search
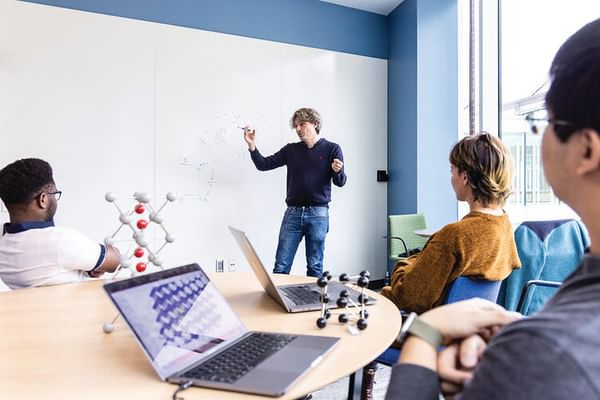
Research Interests
Computational materials design; ab initio computing; high-throughput computing; machine learning; opto-electronic properties of materials; materials for energy production and storage; transparent conducting oxides; thermoelectrics; photovoltaics; high entropy alloys
Education
- MS, Engineering, Ecole Centrale Paris (France) 2004
- MS, Materials Science and Engineering, Université Libre de Bruxelles (Belgium) 2004
- PhD, Materials Science and Engineering, Massachusetts Institute of Technology 2011
Awards
- Chemistry of Materials Reviewer Excellence Award (2018 and 2019)
- Finalist of the Rising Star in Computational Materials Science (2018)
- Marie Curie Fellow (2012)
Professional Activities
- Associate editor, npj Computational Materials
- Group leader for the working group high-throughput screening and data analytics from the psi-k society
- Reviewer for numerous journals (Nature Communications, Journal of the American Chemical Society, Chemistry of Materials, Nature Materials, Joule, Physical Review Letters, Science Advances, …) and government agencies (US DOE, Germany DFG, Swiss National Science Foundation)
- Conference organizer: Materials Research Society symposium (May 2016); European-Materials Research Society symposia (May 2015, 2017 and 2019); IUPAC World Chemistry Congress symposium (July 2019); American Physical Society (2021)
Startups
Matgenix
Founder and CSO
Founder and CSO
Research Projects
-
Searching for New Rare-Earth-Free High-Performance Permanent Magnets
Searching for New Rare-Earth-Free High-Performance Permanent Magnets
There is an enormous need for permanent magnets that do not contain rare earth (RE) elements. However, there is a gap between the performance of non-RE magnets and RE magnets. The aim of this project is to rapidly screen large numbers of compounds that have the potential to be permanent magnets using computational techniques. Both the magnetic saturation and anisotropy are indicators of potential high-performance permanent magnets. Once the compositions of potential permanent magnets are identified, they are cast, the phases present are identified using X-ray diffraction, and the magnetic properties determined.
-
High-strength, high-ductility, high entropy alloys with high-efficiency native oxide solar absorbers for concentrated solar power systems
High-strength, high-ductility, high entropy alloys with high-efficiency native oxide solar absorbers for concentrated solar power systems
This project is investigating the synergy between the excellent mechanical behavior of FeMnNiAlCr high entropy alloys (HEAs) and the high solar absorptance of their native thermal oxides for high efficiency concentrated solar thermal power (CSP) systems working at >700oC. The alloy itself would be used in high-temperature tubing to carry molten salts or supercritical carbon dioxide (sCO2), while the native oxide would act as a high-efficiency solar thermal absorber. The oxide layer is also dense and protective against oxidation (parabolic growth kinetics) at 750 °C. This new Fe-Mn based HEA system has already demonstrated a higher tensile strength and ductility than more expensive Ni-based Inconel 740 superalloys at both room temperature (with carbon doping) and 750oC (with Ti doping), and their native thermal oxides have achieved a high optical-to-thermal conversion efficiency of ηtherm=90.8% at 750°C under 1000x solar concentration ratio. In preliminary corrosion studies, two-phase Cr-modified HEAs have sustained bromide molten salts for 14 days at 750°C with <2% weight loss. The project has close collaborations with the Oak Ridge National Laboratory (ORNL) and the Ames Laboratory in computational materials science and atom probe tomography (APT) to understand the fundamental structure-property relationship in FeMnNiAlCr HEAs.
Funded by DOE.
-
The Materials Project
The Materials Project
By computing properties of all known materials, The Materials Project aims to remove guesswork from materials design in a variety of applications. Experimental research can be targeted to the most promising compounds from computational data sets. Researchers will be able to data-mine scientific trends in materials properties. By providing materials researchers with the information they need to design better, The Materials Project aims to accelerate innovation in materials research.
-
Porous thermoelectric cells (TECs) for waste heat recovery
Porous thermoelectric cells (TECs) for waste heat recovery
Porous thermoelectric cells (TECs) are being developed for waste heat recovery. This project seeks to convert waste thermal energy directly into electricity potentially increasing overall energy efficiency by 15-20% and providing new portable electric power sources, particularly for cold regions. The project is focusing on low cost, nanostructure-engineered TEC (NETECs) materials based on earth-abundant, highly machinable metallic alloys and intermetallic compounds by engineering the grain sizes, second phase precipitation, and nanopores in transition metal intermetallic compounds. The project is currently focusing on the compound Fe2AlV. The location of quaternary atoms in the lattice is being determined by the TEM-based technique ALCHEMI.
Funded by USA-CRREL
-
ECAE processing of Tau-MnAl magnets
ECAE processing of Tau-MnAl magnets
Demand for high-performance permanent magnets is increasing rapidly for applications such as wind turbine generators and motors in both electric and hybrid cars. This market is served by rare earth (RE) magnets based on Nd2Fe14B and Sm2Co17. RE magnets are not without issues; they can chip, suffer thermal shock, and can suffer grain boundary corrosion. However, their biggest problems are: price volatility; that China largely controls the RE metals market; and that the extraction of RE metals creates severe environmental degradation. L10-structured Tau-MnAl has been of interest as a permanent magnet since the early 1960s. It has a theoretical energy product, (BH)max, between that of AlNiCo magnets and RE magnets with a value (12 MGOe) comparable to that of bonded Nd2Fe14B magnets. Further, it does not suffer from the issues associated with RE magnets, and potentially has the lowest cost per MGOe of any permanent magnet. The enigma is that the theoretical (BH)max has never been achieved: mechanically-milled particulates can show high coercivity (HC) but low saturation magnetization (MS) while warm-extruded material can show high MS but low HC. Tau-MnAlis a metastable phase that transforms from the high temperature ε phase, during which anti-phase boundaries (APBs), twins, stacking faults and dislocations are created. Depending on the processing conditions, the equilibrium β and γ2phases can also form. The fundamental difficulty with improving the magnetic properties of Tau-MnAl is that there is no clear understanding on how they depend on the defect structure. The grain size can also influence the magnetic properties either directly or by affecting the β and γ2 arrangement and defect formation.
We are using equal channel angular extrusion (ECAE) to process Tau-MnAl billets over a range of temperatures. Multiple passes will be performed utilizing Routes A (extruded billet is fed back into the ECAE jig in the same orientation) and BC(extruded billet is rotated 90o clockwise about its axis between passes). We will determine the texture of the extruded billets, and the density and arrangement of APBs, twins, stacking faults, dislocations and second phases after each pass, and relate these to the magnetic properties. The local chemistry will be explored at high resolution using atom probe tomography via collaboration with Dr. Baptiste Gault, Max-Planck-Institut fur Eisenforschung, Germany. To extend the strain range and, hence, defect densities studied we will also explore using severe plastic deformation through a collaboration with Prof. Gheoghe Gurau, University of Galati, Romania. Our working hypothesis is that we need a strong, c-axis alignment and a low density of APBs, twins and stacking faults (which locally disorder the material) for a high MS, while a low density of APBs, twins and stacking faults but a high dislocation density are required for a high HC. It is thought that a fine distribution of β and γ2 phases will also contribute to a high HC through magnetic domain wall pinning.
Funded by the National Science Foundation.
Selected Publications
- First-principles study of the T center in silicon, D. Dhaliah, Y. Xiong, A. Sipahigil, S.M. Griffin, G. Hautier, Phys. Rev. Mater. 6, L053201 (2022).
- High-throughput computational search for high carrier lifetime, defect-tolerant solar absorbers, D. Dahliah, G. Brunin, J. George, V.A. Ha, G. M. Rignanese, G. Hautier, Energy Environ. Sci.14, 5057–5073 (2021).
- The Limited Predictive Power of the Pauling Rules, J. George, D. Waroquiers, D. Di Stefano, G. Petretto, G.-M. Rignanese, G. Hautier, Angewandte Chemie, 59, 2–9 (2020)
- Sr3CrN3: A New Electride with a Partially Filled d-Shell Transition Metal, P. Chanhom, Kevin E. Fritz, L. A. Burton, J. Kloppenburg, Y. Filinchuk, A. Senyshyn, M. Wang, Z. Feng, N. Insin, J. Suntivich, and G. Hautier, Journal of the American Chemical Society, 141, 10595 (2019)
- Superionic diffusion through frustrated energy landscape, D. Di Stefano, A. Miglio, K. Robeyns, Y. Filinchuk, M. Lechartier, A. Senyshyn, H. Ishida, S. Spannenberger, D. Prutsch, S. Lunghammer, D. Rettenwander, M. Wilkening, B. Roling, Y. Kato, G. Hautier, Chem, 5, 2450 (2019)
- High-Throughput Identification of Electrides from all Known Inorganic Materials, L. Burton, F. Ricci, G.-M. Rignanese, G. Hautier, Chemistry of Materials, 30, 7521 (2018)
- High-throughput design of non-oxides p-type transparent conducting materials: data mining, search strategy and identification of boron phosphide, J. Varley, A. Miglio, V.-A. Ha, M. Van Setten, G.-M. Rignanese, G. Hautier, Chemistry of Materials, 29, 2568 (2017)
- High-Mobility Bismuth-based Transparent p-Type Oxide from High-Throughput Material Screening, A. Bhatia, G. Hautier, T. Nilgianskul, A. Miglio, J. Sun, H. J. Kim, K. H. Kim, S. Chen, G. -M. Rignanese, X. Gonze, and J. Suntivich, Chemistry of Materials, 28, 30 (2016)
- Unlocking the Potential of Cation-Disordered Oxides for Rechargeable Lithium Batteries, J. Lee, A. Urban, X. Li, D. Su, G. Hautier, G. Ceder, Science, 343, 519 (2014)
- Identification and Design principles of low hole effective mass transparent conducting oxides, G. Hautier, A. Miglio, G. Ceder, G.-M. Rignanese, and X. Gonze, Nature Communications, 4, 2292 (2013)
Patents
Courses
- ENGS 85.09: Introduction to Computational Materials Science and Engineering
- ENGS 137: Molecular and Materials Design using Density Functional Theory
- ENGS 24: Science of Materials
Videos
Accelerated materials design using high-throughput first principles computations (Seminar)
News




In the News
pv magazine International
Scientists identify new ‘promising’ absorber material for thin-film solar cells
Mar 18, 2024
Scientists identify new ‘promising’ absorber material for thin-film solar cells
Mar 18, 2024