- Undergraduate
Bachelor's Degrees
Bachelor of ArtsBachelor of EngineeringDual-Degree ProgramUndergraduate AdmissionsUndergraduate Experience
- Graduate
Graduate Experience
- Research
- Entrepreneurship
- Community
- About
-
Search
All Thayer News
Dartmouth Engineering Study Paves the Way for Accessible Quantum Information Technology
Nov 15, 2023 | by Catha Mayor
Dartmouth Engineering researcher Yihuang Xiong is lead author on a study recently published in Science Advances presenting a new method for identifying promising quantum defects in silicon with numerous applications such as for information technology. The authors used high-throughput computational screening to accurately identify and evaluate a large number of defects with favorable optical and electronic properties.
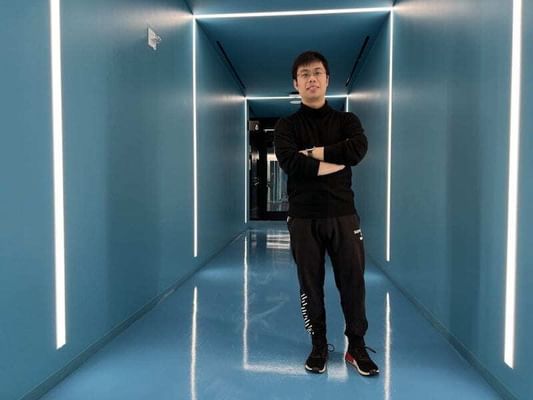
Dartmouth Engineering research associate and lead author Yihuang Xiong. (Photo by Mengxi Song)
"Our work paves the way for accelerated quantum defect discovery and design, aiming to identify which impurities, when introduced into silicon, can create functional and accessible qubits for quantum information technology," says Xiong.
The DOE-funded study, titled "High-throughput identification of spin-photon interfaces in silicon" is a collaboration between Dartmouth and researchers in both Belgium and Berkeley, CA. We spoke with Dartmouth Engineering professor and corresponding author Geoffroy Hautier about the full significance of the findings:
What is the overall goal of this work?
We're helping advance the implementation of quantum information technology which encompasses everything you do with information—computing, sending, and storing—but using the "weird" laws of quantum mechanics. Today's microelectronics are already silicon-based, but we're trying to do something else with silicon.
How are defects in silicon a good thing?
It's interesting—defects are not always negative in materials science. Sometimes, such as for solar cells, you want to get rid of defects and purify your material as much as possible. Other times, such as for certain mechanical properties of alloys, you add defects to make them better. So it depends.
Here, we're helping design certain defects, certain impurities, to our advantage. We're looking at what are called point defects because they're just one or two atoms substituted by another atom. So, for example, you take one atom of silicon and you put titanium instead.
Sometimes you get impurities in silicon because it's just in the environment—like carbon or oxygen, because it's all around us. But you can also put in defects intentionally. We add intentional defects in microelectronics, for instance, for controlling the conductivity of semiconductors.
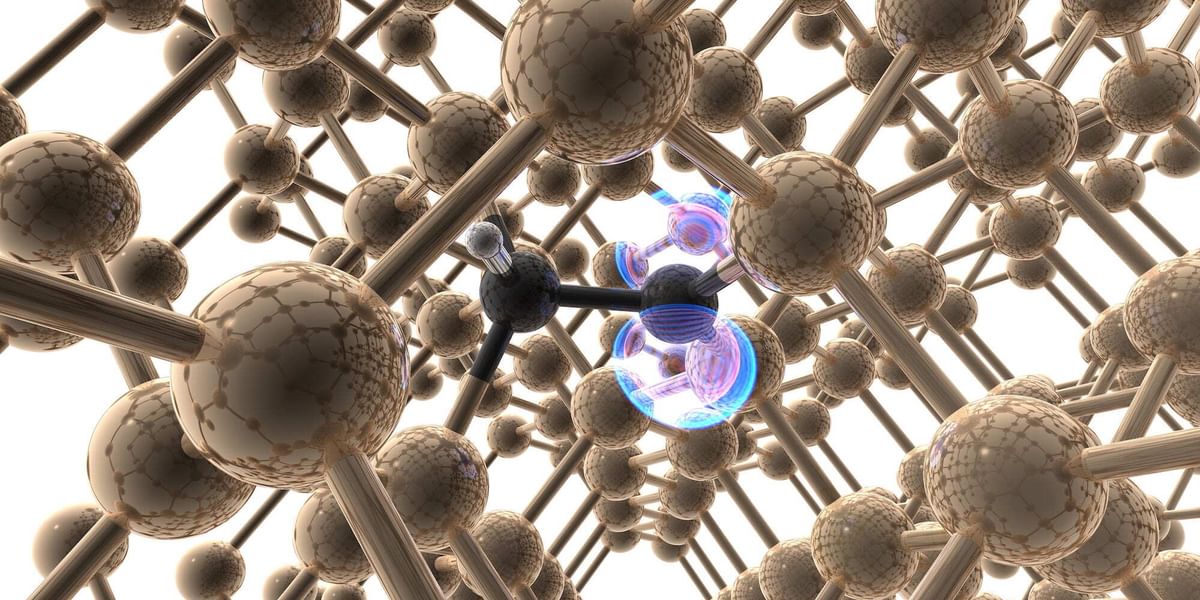
"We're looking at what are called point defects because they're just one or two atoms substituted by another atom." (Image by Weiru Chen and Yihuang Xiong)
Why is silicon the material of choice?
Silicon is an important material because it can be mass-produced and can be extremely pure. So it's a great material for what we're trying to do. There's an established billion-dollar industry that you can rely on. All computers are silicon-based.
So we're screening to see if I add a little bit of something—a minute amount, maybe one atom in 1000—in silicon, can I use this impurity, this defect, to do something related to quantum applications?
What are "quantum applications"?
The idea of a quantum application is to make use of the weird mechanics of matter, such as what's called "superposition." Superposition refers to the way an atomic particle can have undefined or multiple values at once. So it can be, at the same time, both a zero and a one. In standard computing, zeros and ones are called bits. In quantum computing, they're called qubits. But instead of having a specific state like "0 0 1 1," or whatever number, qubits can be in a state of multiple possibilities at once.
This is why, with a well-designed algorithm, you can solve problems much faster. Because instead of saying, "I'm computing this number plus this number," you can compute billions of numbers simultaneously and get billions of results. That's the idea of quantum computing.
Is speed the biggest advantage?
Not for everything, but certain problems can be solved much, much faster. For example, we're simulating how electrons behave in materials, and this can be used to design new materials or new drugs—it can be used for many cool and important things. These problems take a long time on a normal computer. A quantum computer opens an entirely new alley for modeling and understanding matter.
Quantum communication can be also be useful because of the nature of the way you send information. A quantum system can provide the most secure transmission in the world because you can know physically if that information has been read before it reaches you.
For example, if you look at photons that are prepared in a certain quantum state in a specific way, you can actually see if somebody has looked at the photons before you. It's the ultimate security.
And that's the link with what we do here because one of the applications of these silicon defects is especially for quantum communication where computers talk to each other through the emission of light.
Do quantum computers exist now?
There are some now that you can use to solve problems, but they're too small. They have, let's say, ten qubits, but they need 1000 or 1 million. So one of the big challenges is to scale them up, to make them big enough to compete. There's a lot of challenge in doing that and there's a lot of materials science involved in making these technologies better.
How does your work fit into that goal?
What we're doing is mainly related to quantum communication. The field of quantum information science has many branches, among them are computing and communicating—sending quantum information. Quantum communication is related to computing because if you have ten quantum computers, they need to talk to each other. And that's one way we can make them bigger is to have ten different ones that talk to each other.
Is there an AI component to the screening method?
Not yet, but there could be in the future because we're building the data. One of the first steps of this work is building a database of defects in silicon for the specific application of using them for quantum information. We looked at every possible simple defect that you can make in silicon and started deciding, "Okay, this one is interesting. This one is less interesting. Et cetera, et cetera."
Experimentally, it's extremely difficult to test all of that. So we're suggesting what experiments to do, and learning from that—what works, what doesn't work—and improving our model as we go.
Where is this database of defects?
As part of this project, we built a website called "Quantum Defect Genome" at defectgenome.org. It's still under construction. We published the data in the paper, but it's not easy to use. We wanted something easier for people to go through and it will be a cool platform to keep releasing data.
There's something called the Materials Genome Initiative that was started approximately 10 years ago by research agencies to promote the use of informatics and data in materials. The idea is to figure out the genes of materials—what makes a material have certain properties—based on really large data.
Our work is a nice twist on that. We're doing the defect genome, focusing on the defects in the material.
As a materials scientist, did you expect to be working on information technology?
For me it's a natural thing, because the reason we have computers—it's a lot of materials science. It's understanding the physics and chemistry of silicon. How do you etch or remove certain parts of silicon? How do you treat the surface? All these things. Silicon changed the world. All the computing, all the smartphones, all these things are impossible without silicon and materials science.
As traditional microelectronic and information technology has depended on advances in materials science, a similar thing is happening for this new field of quantum information technology. The real-life implementation of quantum technologies will need materials science.
For contacts and other media information visit our Media Resources page.