- Undergraduate
Bachelor's Degrees
Bachelor of ArtsBachelor of EngineeringDual-Degree ProgramUndergraduate AdmissionsUndergraduate Experience
- Graduate
Graduate Experience
- Research
- Entrepreneurship
- Community
- About
-
Search
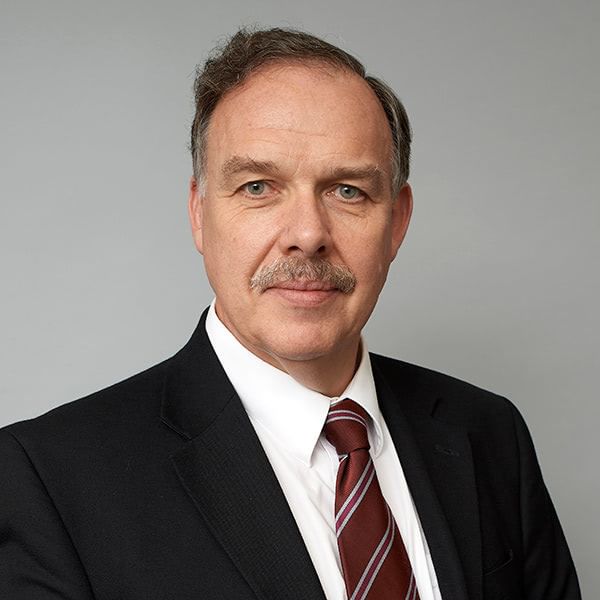
Ian Baker
Sherman Fairchild Professor of Engineering
Senior Associate Dean, Research and Graduate Programs
Baker discusses his research, focusing on the properties and the mechanical and magnetic behavior of materials, and how this work can help power plants be much more energy efficient.
Research Interests
Materials for energy systems; mechanical behavior; phase tranformations; electron microscopy; x-ray topography; x-ray diffraction; microstructure and mechanical behavior of snow, firn, and ice; magnetic materials; intermetallic compounds; high entropy alloys; high temperature materials; nanoparticles for magnetic hyperthermia; biomaterials
Education
- BA, Metallurgy and Science of Materials, University of Oxford 1979
- D.Phil, Metallurgy and Science of Materials, University of Oxford 1982
Awards
- 2015 Fellow, American Association for the Advancement of Science (AAAS)
- 2012 Fellow, Minerals, Metals & Materials Society (TMS)
- 2011 Fellow, Materials Research Society
- 2003 Listed in ISI citation index of highly-cited Materials Scientists
- 2002 Fellow, The Institute of Materials, Minerals and Mining (U.K.)
- 2001 Fellow, ASM international
- 1993 Chartered Engineer (C.Eng.) of The Engineering Council (U.K.)
Professional Activities
- Field-Chief-Editor, Frontiers in Metals and Alloys (2022–)
- Co-Editor-in-Chief, High Entropy Alloys & Materials (2022–)
- Organizer, Physics and Chemistry of Ice, Dartmouth (2014)
- Organizer, MRS Fall Meeting Symposium on Intermetallic-based Alloys — Science, Technology, and Applications (2014)
- Editorial Board, Nano Life (2009–)
- Editorial Advisory Board, Intermetallics (1998–)
- Editorial Board, Metals (2010–)
- Editor in Chief, Materials Characterization (2009–2020)
- Editorial Board, International Materials Reviews (2002–2020)
- Reviewer for various US, Canadian, Hong Kong, Swiss, French, and Australian government agencies and numerous journals
- Consultant to various companies on microstructural characterization and materials processing
- Editor of the proceedings of five conferences
Research Projects
-
Interstitial Strengthening in f.c.c. High-Entropy Alloys
Interstitial Strengthening in f.c.c. High-Entropy Alloys
The aim of the project is to understand both the phenomenology and micromechanisms of interstitial strengthening in f.c.c. high entropy alloys (HEA) and medium entropy alloys (MEAs). The working hypothesis is that interstitials have substantial effects on dislocation behavior (and, hence, mechanical properties) by changing slip from wavy to planar due to an increase in the friction stress (possibly due to short-range order, SRO), or a change in the stacking fault energy (SFE). To that end, the project will: determine the effects of interstitials on the stress-strain curves of single-slip-oriented single crystals, followed by post-mortem examination, using a TEM and an SEM, of the defect structure of crystals strained to various elongations; perform in situ deformation experiments in a TEM and an SEM, and use neutron diffraction to determine the dislocation behavior in detail; determine, using X-ray diffraction (XRD), STEM (including electron diffraction and X-ray spectroscopy), and atom probe tomography (APT), the effects of interstitials on the lattice (the size mismatch parameter, the modulus mismatch parameter, SFE, SRO) and their segregation to dislocations both before and after straining; and determine the exponents in the relationships between the increase in yield strength and both the interstitial concentration and the mismatch parameters. The work will be performed on the HEA/MEAs Fe40Mn40Co10Cr10 and CoCr0.25FeMnNi doped with various interstitials, and, time permitting, Fe40.4Ni11.3Mn34.8Al7.5Cr6. The work will involve synchrotron XRD measurements at the Argonne National Laboratory, and both in situ straining neutron diffraction studies and APT studies at the Oak Ridge National Laboratory.
-
Fabrication of Columnar-Grained Microstructures and Single Crystals via Directional Recrystallization of Additively-Manufactured Nickel-based Alloys
Fabrication of Columnar-Grained Microstructures and Single Crystals via Directional Recrystallization of Additively-Manufactured Nickel-based Alloys
Ni-based superalloys were developed in the 1940s and have been continuously improved ever since, culminating in the current single-crystal jet turbine blades made by directional solidification. However, the cost of such turbine blades exceeds $15,000 and replacement costs when a jet engine is overhauled can be hundreds of thousands to millions of dollars. Thus, a cost-effective method of producing Ni-based superalloy turbine blades is imperative. In this project, we will produce columnar-grained structures or single crystals of nickel alloys by directional recrystallization (DR), a solid-state process, of additively manufactured (AM) material, in which the complex structures are built layer-by-layer by powder fusion. The goal is to investigate if production of a single crystal Ni-based superalloy turbine blade is feasible via the integrated route of AM and DR, and to understand the physics underlying such a processing route. The technology, although focused on Ni alloys, is nonspecific and could be used for other materials, and easily scaled up. We will also develop a web site describing DR of AM materials and develop an undergraduate laboratory project.
-
Searching for New Rare-Earth-Free High-Performance Permanent Magnets
Searching for New Rare-Earth-Free High-Performance Permanent Magnets
There is an enormous need for permanent magnets that do not contain rare earth (RE) elements. However, there is a gap between the performance of non-RE magnets and RE magnets. The aim of this project is to rapidly screen large numbers of compounds that have the potential to be permanent magnets using computational techniques. Both the magnetic saturation and anisotropy are indicators of potential high-performance permanent magnets. Once the compositions of potential permanent magnets are identified, they are cast, the phases present are identified using X-ray diffraction, and the magnetic properties determined.
-
High entropy alloy soft magnets
High entropy alloy soft magnets
Soft magnets play a vital role in efficient energy conversion in a variety of important applications and industries including wide-bandgap semiconductors, electric vehicles, aeronautics, and aerospace, particularly at high temperatures. Improving the efficiency of modern power electronics and electrical machines via advanced soft magnets has the potential to significantly contribute to global energy savings, thereby leading to a reduction of the associated carbon footprint. In this project, we are working on two novel FeCoMnAl high-entropy alloy (HEA) soft magnets, one of which is single-phase B2 (Fe30Co40Mn15Al15) and the other consists of an ordered B2-phase matrix enriched with Co/Al and uniformly distributed BCC nanoprecipitates enriched with Fe/Mn (Fe40Co30Mn15Al15). The two HEAs show similar properties, viz., a high saturation magnetization of 158-162 Am2 kg-1, a high Curie temperature of 1020-1081 K, a low coercivity of 108-114 A m-1, a high electrical resistivity of ~230 µΩ cm, and good thermal stability. We are processing these HEAs using both a powder metallurgy route and via additive manufacturing. The magnetic properties and microstructures of the resulting materials are being examined using combination of a VSM, TEM, SEM and XRD examinations.
-
Biodegradable zinc alloys for orthopedic implants
Biodegradable zinc alloys for orthopedic implants
Orthopedic implants are widely used to treat bone and joint disorders, such as fractures, osteoarthritis, and spinal deformities. However, conventional implant materials, such as stainless steel, titanium, and cobalt-chromium alloys, have several limitations: (i) they may cause adverse reactions and metal sensitivity due to their foreign ions and corrosion products; (ii) they may fail prematurely due to stress concentration and fatigue; (iii) they may interfere with bone remodeling and healing due to their mismatched mechanical properties with the host tissue; (iv) they can become a nidus for bacterial infection and biofilm colonization. Therefore, there is a need for novel implant materials that can overcome these challenges and improve the clinical outcomes of orthopedic surgery. Zinc is an attractive candidate for orthopedic implants because it is an essential trace element in the human body that plays a key role in bone metabolism and wound healing. Moreover, Zn is biodegradable and can be gradually resorbed by the body without leaving any permanent foreign material. The typical in vivo corrosion rates of unalloyed zinc in rats is 0.03 mm/yr, which is a useful rate for biodegradation. However, unalloyed Zn has very poor mechanical strength, which limits its application as an implant material. To address these issues, we are developing new Zn-based alloy that contains small amounts of silver, calcium, iron, magnesium, and manganese, and using a novel processing route. These alloying elements are chosen based on their beneficial effects on the biological and mechanical properties of Zn. Specifically, Ag has antibacterial activity and can reduce the risk of infection; Ca can promote bone formation and integration; Fe can enhance fracture fixation and blood compatibility; Mg can improve biocompatibility and corrosion resistance; Mn can increase ductility and strength. An adequate rate of implant degradation will allow the bone to heal properly before degrading and allowing the bone to support any loads.
-
Observations and micromechanical modeling of the behavior of snow/ice lenses under load in order to understand avalanche nucleation
Observations and micromechanical modeling of the behavior of snow/ice lenses under load in order to understand avalanche nucleation
The microstructual evolution of snow under a temperature gradient has been of interest for many years since this can lead to persistent weak layers, which are possible microstructural causes of avalanches. Ice crusts can form on top or within a snowpack from a variety of meteorological conditions including significant melt/freeze or freezing rain events, and once buried, they can persist throughout the entire winter season and act as an ideal sliding surface for dangerous slab avalanches in seasonal mountain snowpacks. Both of these phenomena are important because the number of fatalities from avalanches in the US has increased annually since the 1970s. Avalanches can also have substantial economic impacts due to road closures, the costs of rescue and building damage, and, with continued global warning, more avalanches are expected in Arctic regions. To understand avalanche nucleation, we are deforming two types of specimens (heterogeneously-layered snow and snow containing an ice lens) in a micro CT located in a cold room, in which the specimens are repeatedly imaged during loading. We are also performing more macroscopic deformation experiments on larger samples at both different rates and different temperatures, which are imaged using a high-speed video camera during loading. The final deformed microstructures in both cases are imaged at high resolution using a scanning electron microscope, which provides information on both the effects of crystal orientation on deformation while clearly delineating one ice crystal orientation from another. Based on the experimental observations, a multiscale computational model is being built to understand crack initiation/crack propagation as well as the deformation mechanisms in heterogenously-layered snow samples containing persistent weak layers and ice/snow interfaces.
-
Using first principles calculations and electro-pulse annealing to design and manufacture low-cost permanent magnets
Using first principles calculations and electro-pulse annealing to design and manufacture low-cost permanent magnets
Demand for high-performance permanent magnets for motors is increasing rapidly for applications such as wind turbine generators and motors in both electric and hybrid cars. Samarium-Cobalt and Neodymium-Iron-Boron Rare Earth magnets are generally used for such challenging applications. While Rare Earth magnets are the best currently available permanent magnets, they are not without problems such as being brittle, suffering from thermal shock, and experiencing corrosion. Further, over 95% of Rare Earths are produced in China and there has been substantial price volatility. Finally, Rare Earth mining has been associated with severe environmental degradation and large energy usage. NiFe, which has been identified in meteorites as the compound Tetrataenite where it transformed from the high temperature (disordered) f.c.c. phase over thousands of years, has magnetic properties comparable to that of Rare Earth magnets. This research award develops new, low-cost, environmentally-friendly NiFe-based permanent magnets, using both quantum-mechanical calculations to predict the effects of alloying with other elements coupled with experiments to verify the effects of these additional elements on the transformation kinetics and magnetic properties. It uses the novel approach of pulsed electrical heating, which has been shown to accelerate transformations. Both women and under-represented minorities will be engaged in the research. The development of novel NiFe magnets will enable production of permanent magnets to be relocated to the USA. As part of the work, the project develops a web site that offers simple virtual experiments to explain to a wide audience magnetism and the materials science of permanent magnets.
The L10-structured compound Nickel-Iron (NiFe) has the potential to replace Rare Earth (RE) magnets at low cost: NiFe has a magnetic anisotropy energy, ku, of 1.3 x 106 J.m-3 and a saturation magnetization m0MS of 1.59 Tesla, which is comparable to that of Nd2Fe14B. In addition, it has good corrosion resistance. The challenge is that the binary L10 compound has a very low transformation temperature from the high-temperature f.c.c. phase of about 320oC that forms on casting and, thus, orders very slowly at temperatures where it is stable. This project combines ab initio quantum mechanical calculations and experimental work to design new L10-structured NiFe magnets with ternary elemental additions. These ternary compounds potentially have a significantly higher f.c.c.-to-L10 transformation temperatures and higher diffusivities than binary NiFe, but have similar saturation magnetizations. Thus, the L10 phase can be produced at higher temperature in short, commercially-viable times utilizing electro-pulse annealing of cold-worked material, which has also been shown to dramatically accelerate recrystallization in NiFe. The TEM-based technique ALCHEMI is used to determine the atom site locations of these ternary additions in the L10 unit cell. This work demonstrates a practical paradigm for designing magnets and leads to new commercially-viable permanent magnet. Commercially, NiFe can be manufactured by continuous electro-pulse annealing of rolls of sheet material or of rods. NiFe is very ductile and can easily be machined into various shapes.
-
High-strength, high-ductility, high entropy alloys with high-efficiency native oxide solar absorbers for concentrated solar power systems
High-strength, high-ductility, high entropy alloys with high-efficiency native oxide solar absorbers for concentrated solar power systems
This project is investigating the synergy between the excellent mechanical behavior of FeMnNiAlCr high entropy alloys (HEAs) and the high solar absorptance of their native thermal oxides for high efficiency concentrated solar thermal power (CSP) systems working at >700oC. The alloy itself would be used in high-temperature tubing to carry molten salts or supercritical carbon dioxide (sCO2), while the native oxide would act as a high-efficiency solar thermal absorber. The oxide layer is also dense and protective against oxidation (parabolic growth kinetics) at 750 °C. This new Fe-Mn based HEA system has already demonstrated a higher tensile strength and ductility than more expensive Ni-based Inconel 740 superalloys at both room temperature (with carbon doping) and 750oC (with Ti doping), and their native thermal oxides have achieved a high optical-to-thermal conversion efficiency of ηtherm=90.8% at 750°C under 1000x solar concentration ratio. In preliminary corrosion studies, two-phase Cr-modified HEAs have sustained bromide molten salts for 14 days at 750°C with <2% weight loss. The project has close collaborations with the Oak Ridge National Laboratory (ORNL) and the Ames Laboratory in computational materials science and atom probe tomography (APT) to understand the fundamental structure-property relationship in FeMnNiAlCr HEAs.
Funded by DOE.
-
Porous thermoelectric cells (TECs) for waste heat recovery
Porous thermoelectric cells (TECs) for waste heat recovery
Porous thermoelectric cells (TECs) are being developed for waste heat recovery. This project seeks to convert waste thermal energy directly into electricity potentially increasing overall energy efficiency by 15-20% and providing new portable electric power sources, particularly for cold regions. The project is focusing on low cost, nanostructure-engineered TEC (NETECs) materials based on earth-abundant, highly machinable metallic alloys and intermetallic compounds by engineering the grain sizes, second phase precipitation, and nanopores in transition metal intermetallic compounds. The project is currently focusing on the compound Fe2AlV. The location of quaternary atoms in the lattice is being determined by the TEM-based technique ALCHEMI.
Funded by USA-CRREL
Selected Publications
- The phase transformation behavior of Mn-Al rare-earth-free permanent magnets, Thomas Keller, Dylan Barbagallo, Natalya Sheremetyeva, Tushar Kanti Gosh, Katherine S. Shanks, Geoffroy Hautier, and Ian Baker, Journal of Magnetism and Magnetic Materials, 587 (2023) 171331. https://doi.org/10.1016/j.jmmm.2023.171331
- The onset of Recrystallization in Polar firn, Ayobami Ogunmolasuyi, Andrii Murdza, and Ian Baker, Geophysical Research Letters, 50 (2023) e2023GL103435. https://doi.org/10.1029/2023GL103435
- Quantitative three-dimensional imaging of chemical short-range order via machine learning enhanced atom probe tomography, Yue Li, Ye Wei, Zhangwei Wang, Xiaochun Liu, Timoteo Colnaghi, Liuliu Han, Ziyuan Rao, Xuyang Zhou, Liam Huber, Raynol Dsouza, Yilun Gong, Jörg Neugebauer, Andreas Marek, Markus Rampp, Stefan Bauer, Hongxiang Li, Ian Baker, Leigh T. Stephenson, and Baptiste Gault, Nature Communications, 14 (2023) 7410. https://doi.org/10.1038/s41467-023-43314-y
- Mechanical Alloying to achieve Accelerated Formation of Equilibrium Phases in Medium Entropy Alloys, Russell Taylor, Youxiong Ye, Wenqian Xu, Si Chen, and Ian Baker, High Entropy Alloys and Materials, 1 (2023) 354–360. https://doi.org/10.1007/s44210-023-00024-w
- Comparison of the sliding wear of single-phase F.C.C carbon-doped Fe40.4Ni11.3Mn34.8Al7.5Cr6 and CoCrFeMnNi high entropy alloys at cryogenic temperature, Aubrey Tang, Ian Baker, Francis E. Kennedy, Hansheng Chen, Simon Ringer, Min Song, and Elliot Alberts, High Entropy Alloys and Materials, 2 (2024) 74–96. https://doi.org/10.1007/s44210-024-00031-5
- FeMnNiAlCr High Entropy Alloys with High-Efficiency Surface Oxide Solar Absorbers for Concentrating Solar Power Systems, Xiaoxue Gao, Edwin Jiang, Andrew Pike, Eldred Lee, Margaret Wu, Huan Wang, Sheppard Somers, Weiyang Li, Geoffroy Hautier, Ian Baker, and Jifeng Liu, High Entropy Alloys and Materials, published 15 April 2024. https://doi.org/10.1007/s44210-024-00033-3
- Additive Manufacturing of Mn-Al permanent magnets via laser powder bed fusion, Thomas Keller, Wuxian Yang, Wen Chen, and Ian Baker, Materialia, 33 (2024) 101978. https://doi.org/10.1016/j.mtla.2023.101978
- Suppression of anti-phase boundary defects in Mn-Al-Ti permanent magnets, Thomas Keller, Dylan Barbagallo, Tushar Kanti Gosh, Natalya Sheremetyeva, Geoffroy Hautier, and Ian Baker, Acta Materialia, 265 (2024) 119646. https://doi.org/10.1016/j.actamat.2023.119646
- Alternating magnetic field guiding system for MNP hyperthermia treatment of deep-seated cancers, Robert V. Stigliano, Ilona Danelyan, Giga Gabriadze, Levan Shoshiashvili, Ian Baker, P. Jack Hoopes, Roman Jobava, and Fridon Shubitidze, International Journal of Hyperthermia, 41 (2024) 2391008. https://doi.org/10.1080/02656736.2024.2391008
- Severe plastic deformation of Mn-Al permanent magnets, Thomas Keller, Gheorghe Gurau, and Ian Baker, Materialia, 38 (2024) 102251. https://doi.org/10.1016/j.mtla.2024.102251
Patents
- High-entropy alloys with high strength | 11530468
- Oxidation resistant high-entropy alloys | 10190197
- Nanostructured Mn-Al permanent magnets and methods of producing same | 8999233
- Joining of parts via magnetic heating of metal aluminum powders | 8444045
- Joining of parts via magnetic heating of metal-aluminum powders | 8172126
- System and method for use of nanoparticles in imaging and temperature measurement | 7994786
- High-strength nanostructured alloys | 7815850
Courses
Books
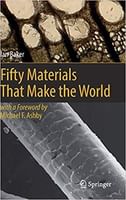
Fifty Materials That Make the World
Springer, 2018
Videos
Graduate Student Engineering Research: Eutectic Alloys
Seminar: Materials Characterization
Lecture: Nanotechnology and its Future Role in Medicine
News




In the News
Solar Power Investor
Dartmouth receives a $750,000 grant from the Department of Energy for a new solar technology research project
Aug 18, 2020
Dartmouth receives a $750,000 grant from the Department of Energy for a new solar technology research project
Aug 18, 2020