- Undergraduate
Bachelor's Degrees
Bachelor of ArtsBachelor of EngineeringDual-Degree ProgramUndergraduate AdmissionsUndergraduate Experience
- Graduate
Graduate Experience
- Research
- Entrepreneurship
- Community
- About
-
Search
All Thayer News
Dartmouth Engineering Contributes to Breakthrough in Understanding of Thin Film Materials
Jul 30, 2024 | by Catha Mayor
A collaboration with a team at UC Berkeley has resulted in a breakthrough not only for a new thin film material for miniaturized electronic systems, but also for our understanding of the electromechanical behavior of thin films overall. The results were recently published in Nature Materials with co-authors including Louis Alaerts, Dartmouth Engineering PhD student, and Geoffroy Hautier, Dartmouth's Hodgson Family Professor of Engineering.
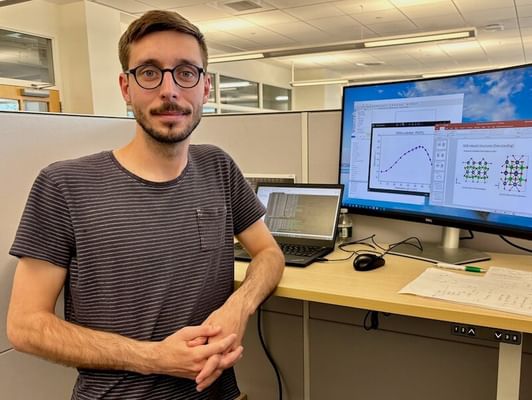
Dartmouth Engineering PhD student and co-author Louis Alaerts.
We spoke with Alaerts in the Hautier Group lab about the collaboration and the resulting advance for materials science:
How did this collaboration begin?
Geoffroy has been working for some time with the PI of this work, Professor Lane Martin [formerly of UC Berkeley and now director of the Advanced Materials Institute at Rice University], so he contacted us about two years ago.
This project is a great example of a collaboration between experimental and theoretical teams. Our group uses computer simulations to predict new materials with interesting properties, and we sometimes help the experimental group to answer the question, "What's going on here?"
What is this breakthrough about?
The ability to miniaturize electronic devices—which is one of the most difficult problems— depends on the performance of very thin films of piezoelectric materials [those that accumulate an electric charge in response to mechanical pressure or movement]. For this, people often use something called lead zirconate titanate, also known as PZT, and the problem is that attaching the piezoelectric film to a substrate—called "clamping"—blocks its movement and inhibits the electromechanical response.
What happened was, the Berkeley team instead used lead zirconate, without the titanium, which people didn't think about doing before because it wasn't a good piezoelectric material in bulk form. When they did that, suddenly the piezoelectric response started working very well.
How did you help explain their results?
We conducted simulations at the atomic level, using the laws of quantum mechanics, to model the material in collaboration with another team at Berkeley—it's good to have two teams working on the same thing to divide some of the tasks and make sure everything is going right.
Mostly, on our side, we looked at the energy barrier to the needed response. When you apply a voltage to the piezoelectric film, it's like little electrical magnets that move to get aligned in the same direction, and that causes a change of shape.
So you have to spend some energy, which is basically the voltage, to get that transition going. And when you add mechanical clamping, there's a chance that this energy barrier becomes too high. This is actually what is happening in other piezoelectric materials such as PZT. So we looked at that and confirmed that the energy barrier wasn't affected by the clamping.
In fact, what we saw was that not only did clamping not reduce the performance, but it seemed to actually increase it. In most materials, stretching in one direction, which is what you want in a piezoelectric, leads to contraction in another. But in lead zirconate, the transition leads to an expansion in all directions, which is very, very unusual. This is actually similar to what happens when you leave a bottle of water in the freezer. That was definitely the biggest and most valuable information the theory team brought.
Why is this important?
Very thin films with a large piezoelectric response have a wide range of applications for miniaturized electronic systems, but it's the basic understanding that drives me.
The material is simple and the concept is simple, but what's valuable is to have all these observations, from both theory and experiments, that provide a microscopic view of the material as it changes shape.
What's next?
We want to continue to improve our understanding of this class of materials, and that's where theory plays a big role.
Current piezoelectrics, including this new material, rely heavily on lead, which is a problem. A better understanding of the mechanism could help us get to lead-free piezoelectric materials in the future.
For contacts and other media information visit our Media Resources page.