- Undergraduate
Bachelor's Degrees
Bachelor of ArtsBachelor of EngineeringDual-Degree ProgramUndergraduate AdmissionsUndergraduate Experience
- Graduate
Graduate Experience
- Research
- Entrepreneurship
- Community
- About
-
Search
All Thayer News
Power Pioneers: Making Tech Cheaper, Smaller, and More Efficient
Jun 02, 2023 | by Michael Blanding | Dartmouth Engineer
Professors Charles R. Sullivan and Jason Stauth Th'00 are leading efforts to make the tech we use every day better—and opening new opportunities in a range of industries.
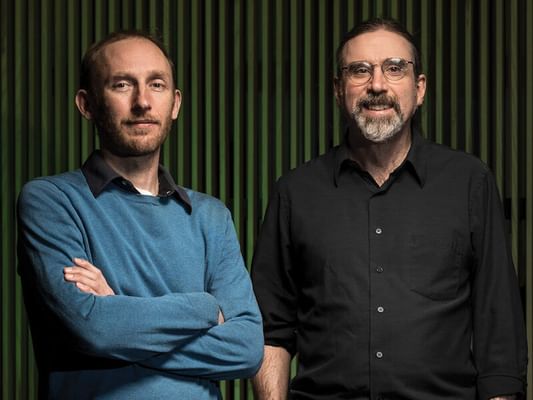
Professors Charles R. Sullivan and Jason Stauth Th'00 (Photo by Rob Strong '04)
Twenty-five years ago, computers were facing a big problem. Microprocessors were using lower and lower voltage to run more efficiently. But to keep up with processing power, they used higher and higher current—and the combination of high current at low voltage began to test the limits of what the power supply could safely produce.
"Intel called that the 'power wall,'" says Professor Sullivan.
So dire was the challenge, in fact, that around the year 2000, experts projected the processors would operate at a thermal density "equivalent to the surface of the sun," says Sullivan's colleague Professor Stauth, and a higher clock rate would cause them to "melt and explode."
Into that crisis stepped Sullivan, who was working on new circuit architectures and components to better transfer power. He created a new design combining a magnetic and an electric circuit, using coupling between inductors—components that store energy in a magnetic field. "That has actually become the standard for high-current power delivery," he says. "It allows the circuit to be more efficient, more compact, and respond fast when the processor load changes."
At the same time, Sullivan helped develop new ways to shrink clunky inductors and transformers so they could be integrated into chips with transistors and other semiconductor devices. This setup allows some processors to run for short periods at high power while others continue running at low power. "You use the high power for only a fraction of a second, while you are loading some complicated webpage or something," Sullivan says. That ultimately solved the crisis. It also enabled the modern computing power of both smartphones and datacenters. "It's a combination of technologies that has prevented that large-scale meltdown."
Sullivan—who will be inducted this summer as a fellow of the National Academy of Inventors—has long been a pioneer in the field of power electronics, or, simply put, the use of electronic components to transfer power. In addition to transforming computing, the field has revolutionized industries including lighting, solar power, robotics, and medical devices—and will be key to creating the new infrastructure for electric vehicles.
"There are a lot of ways of using electricity that don't use power electronics—for example, a traditional coffeemaker is just a heating element that connects to the grid and turns on and off," Sullivan says. "But if you look carefully at what's the best, most efficient technology being used today, it almost always ends up using power electronics."
Sullivan, who has been on the Thayer faculty since 1996 and is currently the Sue and John Ballard '55 TT'56 Professor of Engineering, co-directs the Power Management Integration Center (PMIC), a collaboration between Dartmouth, the University of California San Diego, and 16 industry partners to create technologies for electronics that are increasingly cheaper, smaller, and more efficient. The field, he says, is an exciting combination of concept and practice.
"Sometimes it's very nuts-and-bolts practical and sometimes there's beautiful theory attached to it," Sullivan says. "It's one thing as an engineer when you make something complicated on the bench and it really works—it's another thing when you walk into a room you've never been in before and something you designed is running the lights."
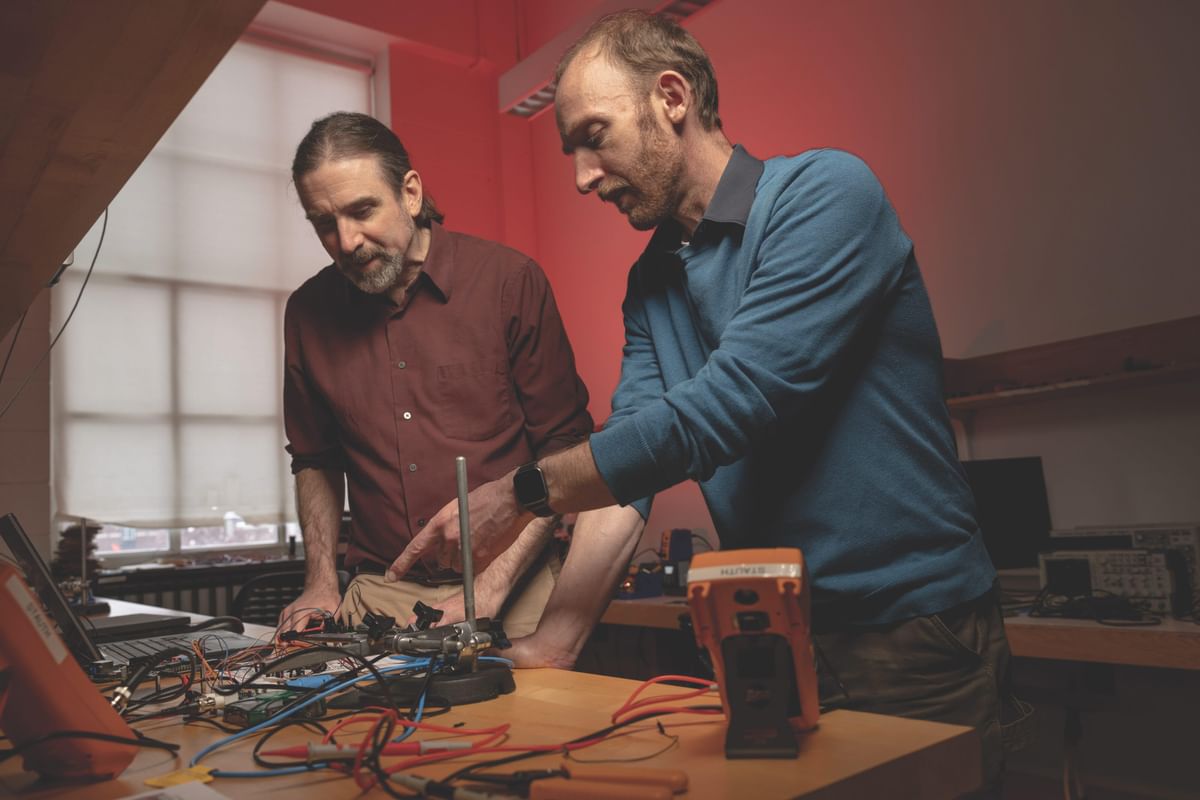
Professors Charles R. Sullivan and Jason Stauth Th'00 (Photo by Rob Strong '04)
Stauth, who co-directs PMIC with Sullivan, has made his own breakthroughs in the field. "I read something 20 years ago that said power electronics is the fastest-growing field in electrical engineering and nobody knows about it," Stauth says about the initial spark that drew him to the topic as an engineering sciences student at Dartmouth. He went on to earn his PhD at the University of California Berkeley before joining the Thayer faculty in 2011.
Soon after, Stauth made a major contribution to the field of solar power by applying power electronics to make photovoltaic systems more efficient. Solar panels use a large number of photovoltaic cells connected in series. "It's like in the old flashlights, where you put a bunch of batteries in series, and if one battery goes bad, it doesn't work," Stauth says. Similarly, in an array of solar panels, if one cell in the array malfunctions—for example, if it is shaded or covered in dirt—"it blocks the power flow, potentially causing problems for the whole array of panels." Stauth developed a technique that moves power around any cells that weren’t working as well so the rest of the array would continue to function.
That technique relied on a new class of power electronic circuits, using something called hybrid-resonant switched capacitor converters. "That’s a mouthful," Stauth allows, but explains it essentially comes down to better ways of using components that passively store energy: inductors, which store it in the form of a magnetic field, and capacitors, which store it in the form of an electric field. While traditional power electronic circuits mainly use inductors as energy storage elements, Stauth realized that using a mix of capacitors and inductors could move energy more efficiently. "We were one of the early groups that recognized the value of merging switched capacitor and traditional, inductor-based circuits," he says. "It turns out the advantage is sizeable."
"A new kilowatt-hour of solar, depending on where you live, is cheaper than almost any other source of energy."
Professor Jason Stauth Th'00
The result has been an increase in efficiency for renewable energy. "At this point, a new kilowatt-hour of solar, depending on where you live, is cheaper than almost any other source of energy," Stauth says. "If you can make solar power more efficient or get more energy per panel, then you are helping to reduce that cost even more." What's more, Stauth's lab extended these circuit techniques to other applications, including electric cars, which are often powered by hundreds or even thousands of connected batteries. Several of Stauth's former graduate students—Eric Din '14 Th'16, Matthew Bossart '14 Th'15, Chris Schaef Th'16, and Keith Moffat Th'16—cofounded Hive Battery Labs, which used related technology to make electric transportation more reliable and efficient.
That transfer into a new application represents how the field of power electronics often evolves, says Sullivan. An engineer comes up with a solution for a specific problem, but the theory often has broader implications. "The specific power electronics design that Jason used for those photovoltaic cells was super-innovative," Sullivan says, "and it seeded a whole new area in the development of power electronics that has now become really popular."
Sullivan is currently pursuing a new project to dramatically improve wireless power transfer, technology that could revolutionize consumer electronics, vehicles, and medical implants. More than five years ago, he cofounded Resonant Link with former postdoctoral research associate Aaron Stein, Grayson Zulauf '12 Th'13, and Phyo Aung Kyaw Th'19. It now has offices in Boston, South Burlington, Vermont, and Zurich, Switzerland.
The technology relies on a totally new type of circuit architecture that changes the way energy moves. "I've been interested in it forever, but we started working on it in earnest when we figured out a way to make a fundamental change in the way it is done," Sullivan says. Typically, energy resonates back and forth between inductors and capacitors, losing a little bit of energy as it sloshes back and forth. That might not matter in a wired electrical system but can dramatically reduce efficiency when dealing with wireless power, Sullivans says. "If you lose even one percent, the efficiency ends up being terrible." To help fix that problem, he and his cofounders created a way to integrate capacitors directly into the magnetic coil of the inductor, combining both components into a single entity.
"Now it can travel just microns apart between those different elements," Sullivan says. "As a bonus, we don't need a separate capacitor," meaning they can make the components smaller as well.
Their new technology could dramatically increase the range of wireless charging by five times. "We're talking one to five centimeters, not 500 miles," he qualifies—but that distance can make all the difference for medical devices that are implanted inside the body. Currently with those devices "either you have surgery regularly to replace the battery, which nobody wants, or you have a wire that goes through the skin, which is uncomfortable." This technology could allow doctors to regularly charge a device inside the body without having to remove it first, reducing stress on patients and opening implants to a wider range of people who need them.
Stauth, meanwhile, is working on a new project involving microrobotics, using power electronics to create small-scale electromechanical systems. These systems use piezoelectric actuators, which require high voltage to provide force or movement, but Stauth's lab has come up with a new way to recycle energy used to drive the actuators, reducing the power needed by more than ten times. This allows the system to run off very small batteries or even on chip micro solar cells. The technology could be used to create tiny robots, such as walking or flying mechanical insects, or small-scale actuators that give sensory feedback when a button is pushed on a smartphone or video game controller.
Looking ahead, one of the more exciting applications of power electronics is in the ongoing revolution in electric vehicles. The technology could be key to not only optimizing battery management within the vehicles themselves but also in transferring power across the vast infrastructure of electric chargers to keep the vehicles running.
"Some of those use really high-power levels that require some pretty intensive power electronics using a lot of the kinds of things we have worked on," Sullivan says. The technology will be essential in charging vehicles quickly on-demand, but the grid can also work in the other direction, using vehicles to return power to the grid when they are not in use. That option makes the whole system more efficient.
In that way, power electronics is helping to make the power grid more sustainable, both by using less power overall and distributing it more efficiently to where it is needed. The more we electrify technology such as vehicles, the more we can rely on renewable energy such as wind and solar to power them rather than polluting fossil fuels. "But once we are using renewable energy, the variability becomes challenging to control and we need all the flexibility we can get," Sullivan says. "Power electronics can give us that control and flexibility we'll increasingly need." As power electronics enable people to live better—with smaller and more powerful consumer devices, longer-lasting lifesaving medical devices, and more efficient electric vehicles—the technologies will be better for the planet as well.
For contacts and other media information visit our Media Resources page.