- Undergraduate
Bachelor's Degrees
Bachelor of ArtsBachelor of EngineeringDual-Degree ProgramUndergraduate AdmissionsUndergraduate Experience
- Graduate
Graduate Experience
- Research
- Entrepreneurship
- Community
- About
-
Search
All Thayer News
Dartmouth Research Team Develops New Method to Measure Neurotransmitters in Real Time
May 01, 2025 | by Catha Mayor
Real-time monitoring of neurotransmitters is key for advancing both basic neuroscience and treatments for brain disorders. A new study published in Nature Communications presents a unique carbon coating for sensing devices to achieve fast electrochemical measurements of neurotransmitters in the human brain.
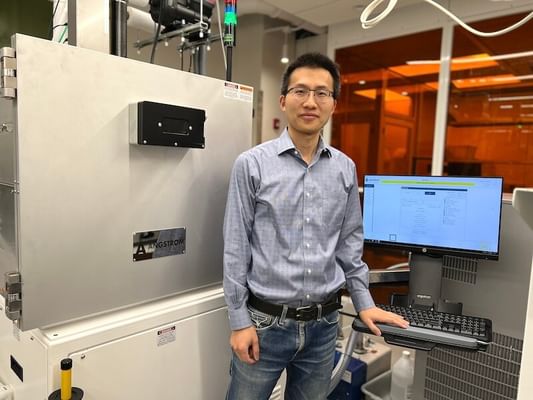
Senior author Hui Fang in his research group's Multifunctional Integrated NeuroElectronics Lab. (Photo by Catha Mayor)
"Neurotransmitters are the messengers in our brain," said Hui Fang, associate professor of engineering and senior author on the paper. "There's only a handful of ways to measure them, all with severe limitations. Traditional microdialysis, for example, is slow—literally pumping liquid out of the brain to measure it externally. So you can't do real-time measurements."
The study's co-authors—a collaboration of researchers from Dartmouth Engineering and the Department of Psychological and Brain Sciences, and Weill Cornell Medicine—focused on the electrochemical measurement method using special electrodes. "In a nutshell, you have a specific electrode, and then you drive electrical potential around it, which causes a chemical reaction between the neurotransmitter and the electrode," explained Fang. "That chemical reaction lets you see and measure the electrical current, and from the current-voltage potential relationship, you can tell what and how much neurotransmitter you're sensing."
Although this method relies on there being a chemical reaction—not the case for all neurotransmitters—it does work for dopamine, serotonin, epinephrine, and a few others which are involved in common mental health conditions such as anxiety, depression, and mood disorders.
The challenge is around what material to use for the electrodes. Carbon fiber has been the gold standard and is reliably sensitive and inert, but it's fragile and hard to scale up and integrate with recording interfaces.
"Our paper addresses these pain points," said Fang. "The ability to scale up this type of sensing to many electrodes in a high-density array that covers a bigger area with higher throughput, and then also to easily integrate them with neural recording—that's a big deal. So we developed a stabilized carbon coating to go on top of the conventional metal electrodes used for electrical signal recording."
During testing, the team discovered that a simple thermal treatment of their coating caused an unexpected and significant improvement in both stability and reliability.
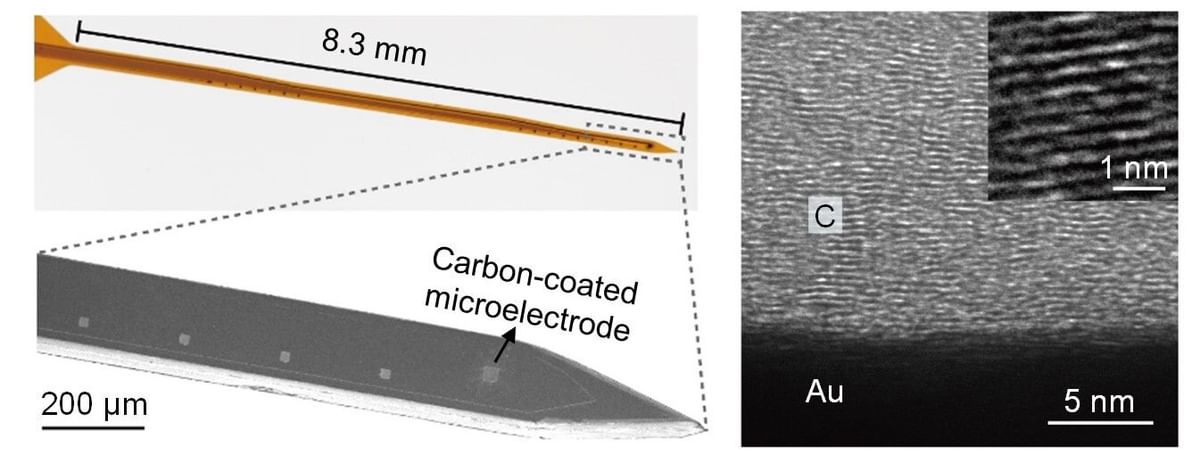
Left: Neural probe with integrated neurochemical sensor and electrophysiological recording sites; photo (top), SEM image (bottom). Right: Transmission electron microscopy image of a cross-section of the carbon-coating following 250 °C thermal treatment.
Their discovery makes it possible to finally marry two worlds together—the chemical sensing world and the electrical activity sensing world, and do them simultaneously.
"This is where we collaborated with Professor Matt van der Meer in Psychological and Brain Sciences," added Fang. "We looked at dopamine signaling to see if there's any correlation with electrical activity, particularly in the so-called high-gamma band, and found there is indeed a strong correlation."
Although not too surprising, the correlation between chemical and electrical activity in the brain has been lacking direct evidence and specifics, until now. This new window on electrochemical activity could help explain the results of deep brain stimulation studies, advance our understanding of brain pathology, and reveal key biomarkers of different brain states.
"The brain is complicated and full of these electrical and chemical activities and signaling," continued Fang. "That's why I'm excited by this work, because the impact is potentially enormous."
For Fang's research group, the next steps are clear. They were recently awarded $2.6M from the NIH over five years to develop and optimize microelectrode arrays for in vivo neuronal sensing and electrophysiological recording. Said Fang, "Refining and validating this type of probe would directly enable numerous studies in both basic and translational neuroscience, would be applicable to many other devices, and would bring the technology a significant step closer to commercial manufacturing."
For contacts and other media information visit our Media Resources page.