- Undergraduate
Bachelor's Degrees
Bachelor of ArtsBachelor of EngineeringDual-Degree ProgramUndergraduate AdmissionsUndergraduate Experience
- Graduate
Master's Degrees
Master of EngineeringMaster of Engineering ManagementMaster of ScienceDoctor of Medicine-MEng Joint DegreeDoctoral Degrees
Doctor of PhilosophyPhD Innovation ProgramDoctor of Medicine-PhD Joint DegreeGraduate AdmissionsGraduate Experience
- Research
- Entrepreneurship
- Community
- About
-
Search
Transcript
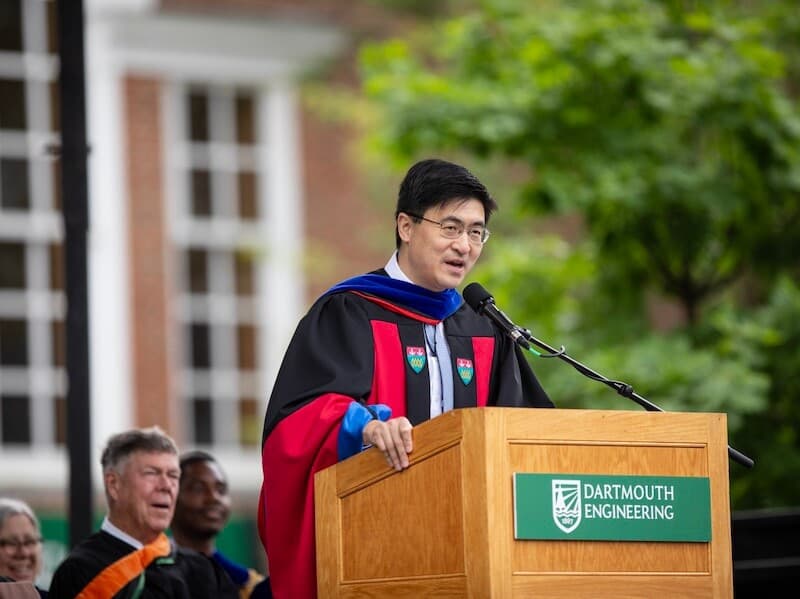
Thank you, Dean Abramson, Provost Kotz, and Trustee Chair Lempres, for the kind introduction and precious opportunity.
Good morning to distinguished colleagues and guests. And congratulations to the Dartmouth Class of 2024 from Thayer! You endured COVID-19 and you are here today: a special and warm celebration for all of you and your families.
I have no idea why this remarkable institution has bestowed on me such an incredible award. What a humbling honor for me, not only because of the pioneering footprints of the awardees in the decades past, starting with Gordon Stanley Brown the year before I was born, but also because of the long and illustrious history and the unique character of Dartmouth's engineering school throughout the past 153 years, the first 47 of which were led by Bobby Fletcher.
In 1936, the Dartmouth Alumni Magazine, in memory of Fletcher, quoted some of his many students, including this one: "My pleasantest memories of the Thayer School are linked with your classes in mechanics, not just the subject matter but of the human interest you gave the subject and the enthusiasm you inspired with it."
In recent years, your innovative faculty, staff and students have further strengthened this human-centered learning and discovery, which has long been part of the institutional DNA, through the cornerstones of systems and design thinking. In honor of Thayer's tradition, I seek your permission this morning to indulge in the nerdy topic of "the characters of engineering design."
Now, it's notoriously challenging to deliver investiture remarks that are fresh and fitting. And in a society infused with tech products, from chips and drones to AI and EV, it is doubly hard to say anything new or unique about engineering. Much has been articulated about the mathematics and the physics of engineering; how about the philosophy of engineering? In particular, wouldn't it be fun and curious to explore recurrent themes and ideas for engineering synthesis? Such recurrence is particularly useful in our age when the artifacts of engineering dazzle and proliferate.
In so exploring, I'll cite examples closer to my specialty areas, but the thematic reach is hopefully broader. I'll try to be brief with each of the three characters, as I'm likely preaching not only to the choir but also to the experts!
1. Trade-offs
Let's start with a viewpoint that engineering synthesis is constrained optimization: There's an objective and there're constraints on design variables. Conversely, every sustained design is optimizing something under some constraints. You can reverse engineer it to better understand how to reengineer it.
But there's something more. Engineering is an art of trade-off among multiple objectives. A multi-objective, or vector, optimization is also sometimes scalarized by moving all but one objective into constraints and progressively relaxing such constraints to visualize the trade-off. This leads to the crucial concept of Pareto optimality: Even when you have multiple objectives, there still is the well-defined notion that a certain configuration cannot be made better in one objective without making another objective worse. In work as in life, that's a powerful way to think about competing goals.
Among the many objectives is yield, a manufacturing metric about the percentage of successfully made products under cost constraints. The technology that proved feasibility may not be the one to sustain scalability. For example, in semiconductor and in pharmaceutical manufacturing, it's inadequate to be able to make the designed product once or twice. It's essential to have a sufficiently high percentage of products that pass the quality test among all that are made. Making, say, one next-generation chip with 3nm feature size is not easy, but making many of them with a high yield is the real challenge.
Speed is another tricky objective to trade off against. Engineering systems often need to react fast enough, either because there are strict deadlines, like I have six minutes left, or because utility decreases over time, as the audience of an investiture speech can attest. But there's also the speed of rolling out and changing a system: that's the agility of design. A perfect but late or stale solution is rarely good enough in engineering.
2. Modularization
Another fundamental idea to synthesis is modularization, which helps to scale in complexity, size and adaptability. Architecture is the study of modularization: "who does what, at what timescale, and how to glue them back together." It's like the CEO's job in a large company: not about how to do each department's work, but how many departments there should be and how they can be connected. We often worry about resource allocation, but a first-order concern is actually functionality allocation.
Essential to the concept of modularization is "interface definition": how would the modules connect to each other in some standardized manner? When the interfaces are properly defined, modularization ensures both optionality and extensibility.
One more powerful feature in modularization: Feedback is a particularly important notion found in nature and used by humans. You may not need to pass a lot of information across the interfaces, but negative feedback, such as pricing signals, is among the most commonly deployed mechanisms to connect modules and stabilize a complex system.
Among the many famous examples of architectural choices is Shannon's information theory in the 1940s: The job of removing redundancy through data compression and the job of adding redundancy to correct against noise can be separated, without loss of optimality in certain baseline cases, and with a standardized interface called digital communication.
Another architectural statement was made by Cerf and Kahn exactly 60 years ago in the TCP/IP architecture for packet switched networks: the job of finding end-to-end paths is separated from both the applications and the physical media, in what is known as the network protocol stack. Their architectural design laid the foundation for the incredible growth of the internet in scale and in scope. Just imagine how untenable networks would have been otherwise, during the past decades of many changes to the applications we use and to the internet connectivity technologies!
3. Virtual-physical-human interactions
The third and final character I'll highlight is arguably a special case of modularity: the trifecta of virtual-physical-human interactions.
Engineers solve problems with atoms, bytes, and people. First, let's consider where atoms meet bytes at the physical-virtual interface. The physical implements the virtual, and the virtual models and controls the physical. For example, you can explore "physical AI:" how might the bytes of AI change the physical world, such as agriculture, manufacturing and transportation?
An interesting philosophical and economic choice arises: Should the virtual-physical interactions be domain-specific, or can they stay generic? In the past, you've seen the contrast between hardware-software co-design by Apple and the alternative of separating the hardware from the operating system.
We also appreciate the distinction between the "integrated device manufacturing" model by some semiconductor companies versus the "foundry model" where chip design companies are fabless and others specialize only in manufacturing. A question right now is whether AI products should be generic, or does it help to be domain-specific? There is no "one size fits all" answer, and in decades to come you might be driving the iterations between these two choices.
Now add the human interactions with the virtual-physical: humans both as objectives and as constraints, both as end users and also as operators and designers. That leads to "simplicity" as an overarching theme of engineering synthesis. Simplicity is the root for robustness, especially when humans operate and redesign engineering artifacts. Simplicity helps make the volatile less brittle.
Conclusion
With these worldviews and systematic approaches, engineering is, in some sense, a language for thinking. I used to be an engineer too, but now I specialize in watching basketball games and sitting in budget meetings, so thank you for being so patient with my nostalgic rant.
Like many of you, I had my intellectual curiosity shaped by faculty advisors. Stephen Boyd and Tom Cover at Stanford taught me not just proofs and experiments but also asking questions and connecting dots so patterns emerge out of fluctuations.
They also taught me that much of what's covered in classes and in speeches will soon be forgotten. What is uncovered by yourself lasts longer. In that sense, your lifelong learning is like a beautiful journey without a destination. And it continues on Monday morning, with the enthusiasm that Fletcher's student remembered.
But in the meantime, enjoy your well-earned commencement weekend with friends and family, and congratulations to the new Dartmouth graduates!