- Undergraduate
Bachelor's Degrees
Bachelor of ArtsBachelor of EngineeringDual-Degree ProgramUndergraduate AdmissionsUndergraduate Experience
- Graduate
Graduate Experience
- Research
- Entrepreneurship
- Community
- About
-
Search
All Thayer News
Dartmouth Engineers Help Develop New Way to Understand Long-Term Damage Following Traumatic Brain Injury
Dec 11, 2024 | by Catha Mayor
A new study shows how the combination of three advanced technologies can reveal the metabolic interactions between human brain cells. The study, published today in Science Advances, presents a novel multidisciplinary platform that could lead to improved understanding, and improved treatment, of the damaging metabolic changes that often follow traumatic brain injury (TBI) and lead to long-term neurodegeneration.
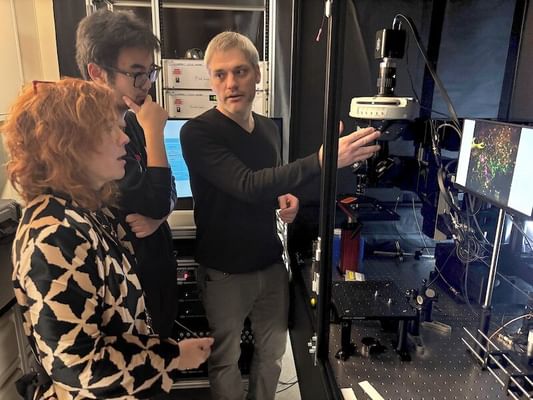
(l to r) Professor Georgakoudi with study co-author and PhD candidate Yuhang Fu, and Research Scientist Matthew Lindley in Dartmouth Engineerings' Optical Diagnostics for Diseased and Engineered Tissues Lab. (Photo by Catha Mayor)
The researchers from Dartmouth Engineering, Tufts University, the University of Pittsburgh, Johns Hopkins, and others, combined an engineered 3D human brain tissue model with non-invasive, high-resolution fluorescence imaging, and studies of microRNA—molecules that help cells control what proteins they make. The combination of technologies could shed new light on how specific biochemical interactions between neurons and glial cells are altered by TBI.
"Preventing neurodegeneration after TBIs could be a game-changer for athletes, soldiers, and anyone else at high risk of multiple brain injuries, even when they are mild—it's an issue that affects many lives," said Irene Georgakoudi, professor of engineering at Dartmouth and senior author on the study. "We want to know how the damage is propagated and if there are pathways that can be targeted to intervene. We focused on metabolism and were able to combine our non-invasive, high-resolution fluorescence imaging with microRNA studies to get a much more nuanced picture of how neurons and glial cells use different metabolic pathways in response to injury."
What makes the imaging technique used in the study non-invasive is that there's no need to slice the tissue thinly to examine it. Instead, unique natural interactions between light and tissue are detected from distinct locations in the 3D model. The method is also label-free, meaning it uses molecules already present in the cells as a source of signal rather than injecting a contrast agent. This enables the researchers to take multiple images of the same specimen over 72 hours, and therefore get a more accurate understanding of how things evolve over time.
"The problem is, although label-free fluorescence imaging on its own can show detailed cellular metabolic changes, it lacks molecular pathway specificity," she explained. "So we analyzed the microRNA data in a way that focused on metabolic pathways to gain these insights."
Added Georgakoudi, "For people who are studying metabolic function in the context of disease, we're showing that you can think about combining label-free imaging tools with techniques like microRNA measurements to really enrich the information you have available and enable real-time monitoring."
The problem of neurodegeneration was chosen for the study because investigating the human brain is especially challenging. Previous studies have shown that metabolic function plays a role in how damage propagates after TBI, but the specifics of the biochemistry and the exact sequence over time remain unclear.
To build on this study, the researchers plan to investigate the impact of multiple injuries of different severity using multimodal AI fusion models that can combine the data even more effectively, and hopefully reveal treatment possibilities to mitigate the metabolic damage.
"And because metabolic dysfunction is relevant to many different diseases" she continued, "we'll be trying the combination of optical imaging and genome analyses for different applications, including cancer."
For contacts and other media information visit our Media Resources page.